In this article, we will take a look at the impact of various sources of pollution on an organism’s ability to detect polarized light.
Written by: Shreya

Our entire lives are dictated by light. From determining the stopping distance to a traffic light to recognizing a familiar face to generating a cognitive map of our homes, photoreception– or vision– enables us to comprehend our surroundings. However, the light that we perceive does not compose light in its totality. Not only are our eyes only capable of registering visible light, which constitutes a tenth of a trillionth of the entire electromagnetic spectrum, but they are also limited to solely discerning unpolarized light. The polarization of light involves the electron field being restricted to a single plane, making the resulting light wave oscillate in that same plane. To species with the capacity to detect it, the polarized nature of light hints at the location of its light source (ie. the sun), can act as a private communication channel between organisms, sets an axis of directionality, and enables long-distance migration (1).
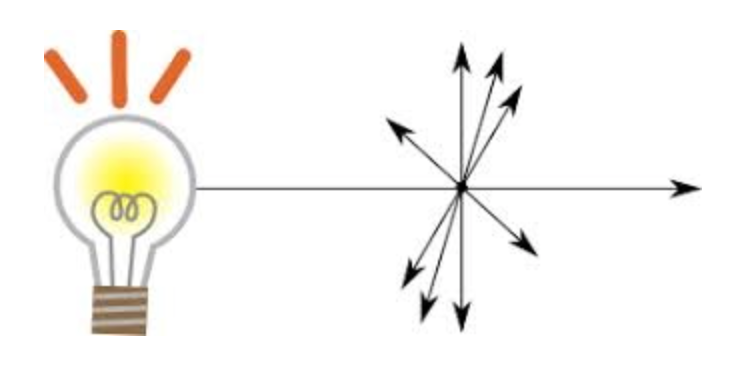
Normal light scatters in all directions; however, in polarized light, the electron vector only points within the same plane.
Normally, air or water molecules scatter unpolarized sunlight into set polarization patterns– a phenomenon known as Raman scattering. These natural patterns of polarization differ throughout the day since their orientations depend on the angle of light directed by the sun. Several species, including many invertebrates such as insects and crustaceans as well as a few vertebrates such as fish, can register the oscillation plane of the light vector and its angle from the horizon in order to discern the time of day and establish their directionality. Thus, by detecting the amount of light reflecting off an object or the intensity of polarization, many animals can use polarized light in navigation, spatial orientation, and time judgment. The rainbow trout, for instance, uses polarized light to navigate underwater, but it does so in a unique way. In their youth, the fish can detect polarized light that penetrates shallow water from the bottom half of their eyes, but as they develop further, their polarized light detection switches to the top half of their eyes so that they can navigate deeper waters while still using the underwater light field (2). Several birds combine their sensory detection of magnetic fields and polarized light to navigate long distances. Although the exact sensory mechanisms behind their migratory abilities are not known, it is predicted that a type of photoreceptor in their eyes, called cryptochrome, enables them to follow magnetic field lines based on the e-vector of the absorbed polarized photons. Studies have even shown that when light is controlled to follow an irregular polarization pattern, birds entirely lose their ability to detect directionality (3).
In addition, several organisms manipulate the oscillation angle of light (known as the e-vector) to privately communicate with other members of their species, preventing predators from gauging their location while signaling messages intraspecially. For instance, the California market squid can change the blaze angle of polarized light by moving or shielding reflective and polarization-sensitive proteins in the cytoskeleton of its skin cells in order to communicate with other squids. The mantis shrimp possesses an especially rare ability: the detection of circularly-polarized light. Most organisms detect linearly-polarized light, where light oscillates in a single plane, but circularly-polarized light consists of two light waves simultaneously oscillating perpendicular to one another, generating a spiral pattern.

Mantis shrimp are extremely territorial and display circularly polarized light patterns on their armored backs and legs when disputing over resources with other mantis shrimp. Studies have shown that when a mantis shrimp is put into a tank with two burrows– one emitting non-polarized light and the other emitting polarized light, the shrimp almost always chose the non-polarized burrow under the assumption that the other burrow was already occupied (4).

Although natural light polarization can greatly aid species through a plethora of tasks, anthropogenic sources of or alterations to light’s polarization can be immensely damaging. These sources can alter breeding, navigation, spatial orientation, and communication, resulting in as dramatic an effect as wiping out an entire population of a species in a region. Take, for instance, the impact of outdoor light fixtures on mayfly oviposition, or egg laying behavior. Mayflies normally detect water bodies in which to breed and lay their eggs through the polarized light refracted off their surfaces. However, the light from outdoor fixtures reflects off asphalt and causes many mayflies to instead lay their eggs on the road, bridges, or cars, leading to the potential decimation of their entire next generation within a single night (5). Several insect species have also lost their sensitivity to light polarization due to interference from anthropogenic light sources that dim polarized skylight signals. Light pollution from urban regions that extends to coastal regions has been found to distort sand hopper (a nocturnal crustacean species) orientation by dimming lunar and stellar visibility that the organisms would normally use to navigate across the beach (6). In fact, night-time light pollution can cause a 62% decrease in pollination visits by nocturnal insects.
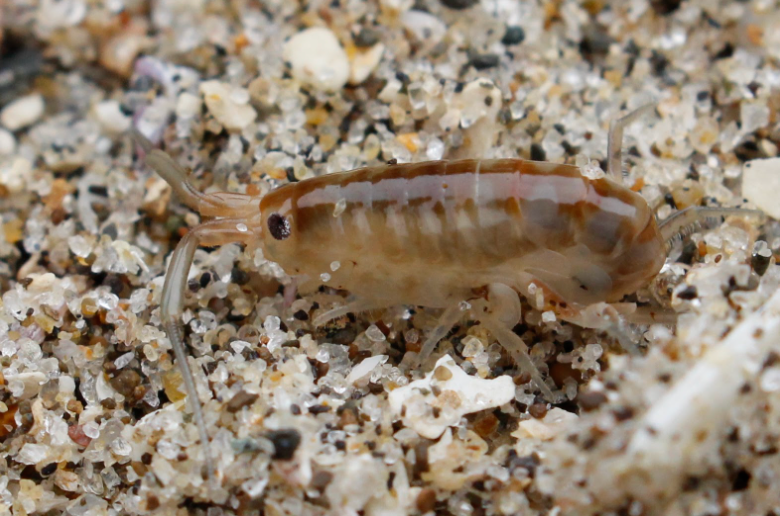
In addition, we took a look earlier at how polarized light plays a role in the magnetoreception capabilities of birds. According to the ‘radical pair” theory, very specific e-vectors of polarized have the ability to excite electrons in the cryptochromes of bird eyes, and the excited electrons generate a spin that then aligns in certain orientations in accordance to earth’s magnetic fields. This alignment is what generates a visualization of field lines, allowing birds to migrate long distances. However, several anthropogenic radiation sources can interfere with these natural polarization patterns, disturbing birds’ senses of directionality. In a study conducted on European robins, it was found that when birds were subjected to a 1.315 MHz field, they were able to follow magnetic fields normally if the radiation oscillated in a plane parallel to the field lines but were completely thrown off course when the MHz field aligned 24 degrees off field lines (7). Thus, if radiation is polarized and oriented to a plane besides that off the geomagnetic lines, the magnetic compass birds use to navigate could break down. Threats may include AM radio waves, lights on communication towers, and radar.
Finally, another source of polarization disruption comes from pollution in the air and water. Air particulates such as soot scatter light irregularly from air molecules and disrupt natural polarization patterns (8). Aerosols, or solid particles suspended in the air such as haze or smoke, also distort skylight polarization trends by decreasing the magnitude of the e-vector (9). Similarly, pollutants in water such as oil, heavy metals, and chemical waste can induce a disarray in the angle of the oscillation plane.
In a society that is constantly swimming in light and radiation, we must remember to consider the impacts of our electromagnetic radiation pollution on organisms that use such lightwaves for survival and reproduction. Frequencies that do not impact navigation and communication should be studied, and night-time light fixtures with the slightest intensity possible should be used. To uphold the biological functions of those with the unique ability to detect light’s polarization, pronounced changes in light consumption, microwave emission, air pollution, and water pollution are needed.
References:
Mantis Shrimp: Communication using a secret, polarized light language
The Devastating Role of Light Pollution in the ‘Insect Apocalypse’
The impact of artificial light at night on nocturnal insects: A review and synthesis
Magnetic compass orientation of migratory birds in the presence of a 1.315 MHz oscillating field
Differentiation of soot particulates in air using polarized light scattering method.
Image References:
Comments