The Impact of Pollution on Sensory Reception: Magnetoreception
- ecure
- Oct 27, 2020
- 5 min read
Updated: Oct 28, 2020
Written by: Shreya
We perceive our surroundings in the minuscule interim between the micro- and macroscopic happenings of the universe. Both the subatomic interactions gluing matter together and the large-scale forces directing our planet’s catapult across the cosmos evade our detection. However, there exists one particularly mysterious form of sensory reception that coagulates both into the comprehension of magnetic field lines: magnetoreception. Large, magnetoreception is the biological ability to visually perceive earth’s magnetic field lines, aiding in long-distance migration and orientation. The biomolecular mechanisms of this sensory capability are largely unknown, with several theories as to how field lines could be translated into action potentials to the brain remaining unanswered, although two anatomical mechanisms in particular have proven to harbor a role: magnetite and cryptochrome.

Magnetite:
Magnetite, or iron oxide, is a naturally-occurring magnetic compound found in the upper beak of several species of birds. Earth’s magnetic field aligns magnets so that the magnetic south pole aligns with Earth’s north pole and vice versa, meaning that a magnetic compound like magnetite would orient itself along magnetic field lines. It is theorized that as birds migrate across the sky, Earth’s variable field lines tug magnetite into a favorable orientation, and this constant reorientation mechanically controls ion channels that biologically relay the direction (north, south, east, west) in which the bird is flying. However, a compass is not enough to signal a bird how to travel hundreds of miles to and from its home with exact precision– for that, a magnetic “map” is needed, and cryptochrome could provide just that.
Cryptochrome and the Radical Pair Theory:
A molecule present in the photoreceptor cells of bird eyes, cryptochrome has been found to play an immense role in magnetoreception by visual light, and scientists have encapsulated its theorized mechanisms in the “radical pair” theory. The photo-excitation of an electron in flavin adenosine diphosphate (FAD), a sub-particle in the cryptochrome complex, triggers the energized electron to transfer over to a nearby tryptophan (TRP) residue. This simultaneously generates two radicals, or molecules with an unpaired electron: the now positively-charged FAD and the now negatively-charged TRP. Radicals engendered together are known as “radical pairs”, and the interactions between their lone electron spins is guided by the external magnetic field. Electrons with the same spin (meaning the same directional orientation) are in the “triplet state”, while electrons with antiparallel spins are in the “singlet state”. Electron pairs oscillate between the two states, and depending on the orientation of their spins with respect to the magnetic field lines of earth, their oscillation takes on certain patterns.

Due to their charge and angular momentum, electrons generate a small magnetic field of their own, and if these fields align with the orientation of their nucleus’s spin (or nuclear spin), they possess a high energy level. On the other hand, electrons with fields antiparallel to the nuclear spin are low-energy. This energy difference is amplified when considering that nuclear spin is aligned with the spin of earth; thus, the energy of an electron field antiparallel to that of earth’s is reduced by the energy of both its nuclear spin and earth’s spin while the energy of a parallel field is boosted by those same values. The energy differences further change when electron orientations occur at an angle to earth’s fields rather than as directly parallel, yielding a strong and direct translation from magnetic field lines to electron energy levels. By registering the difference in energy levels between the electrons in a radical pair, an organism could potentially decipher the angle of the magnetic field line.
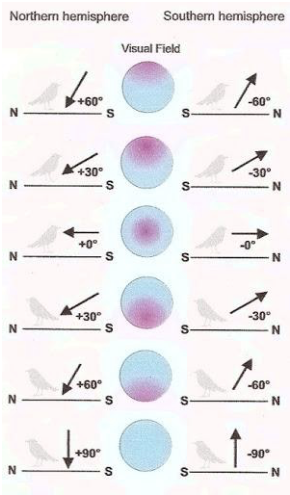
Now that we understand how these field lines could be quantified into energy differences, we can take a look at how the radical pair theory translates this variance into a biological cue. Researchers postulate that as the electrons oscillate between the singlet and triplet state in response to their angle with Earth’s field lines, at least one of the states triggers the release of a biomolecule. The amount released depends on the duration of the state (which depends on the energy difference between the two electrons), lasting for a maximum of 100 microseconds, and the duration directly correlates to the angle with the field. Thus, the presence or absence of this hypothesized molecule and the duration of its presence/absence would be indicative of the magnetic field line’s orientation with respect to the electrons. From there, the molecule released could trigger a signal transduction pathway that amplifies its signal and instigates an action potential, delivering its message to the brain for interpretation. So ultimately, this theory states that the photo-excitation of cryptochrome electrons generates radical pairs that interact with magnetic fields to relay field line orientation to the brain in the form of a biomolecule.
What stuns researchers even more is the possibility of these lone electrons being quantumly entangled, meaning that the spin of one electron is directly dependent on the spin of the other (regardless of the distance or even time separating them). In other words, the quantum state (spin) of one electron cannot be described without knowing the state of the other. So the next time you call someone a “bird-brain”, know that the avian mind might just physiologically be able to understand quantum mechanics better than even the best of humanity’s physicists!
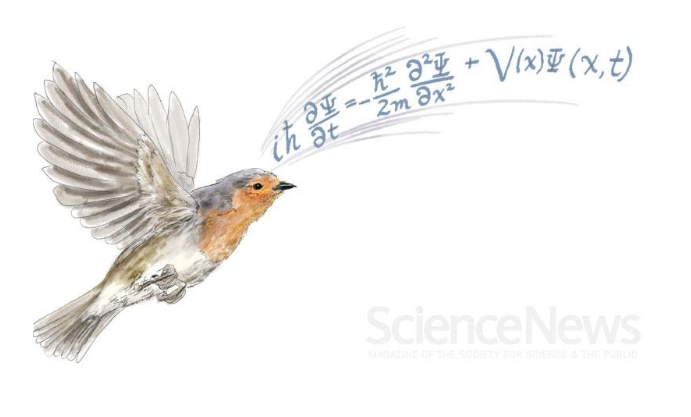
Anthropogenic Threats to Avian Magnetoreception:
Clearly, birds have been found to possess an intricate and mysterious navigation method whose mechanisms have thus far eluded us. However, anthropogenic sources of electromagnetic waves (known as electromagnetic pollution) could potentially threaten avian magnetoreception by disrupting natural light patterns used by cryptochrome to decipher field lines. The Melatonin Hypothesis states that extremely low-frequency magnetic fields (ELMFS) such as those produced by television radiation or electrical lines decrease melatonin production, which increases the risk of cancer. Although much evidence for this hypothesis has not been found on humans, there have been studies on cattle calves and mice that show a relationship between ELMFS and circadian rhythm disruptions; in essence, melatonin levels are seasonally dependent, but ELMFS derange the natural cycle. The consequences of these studies on magnetoreception rest in the fact that cryptochrome is involved in circadian rhythm maintenance. Cryptochrome is blue-light sensitive (which is why photo-excitation triggers its activity), and ELMFS have been shown to alter day-night distribution patterns by shortening the circadian rhythm expressed by the Cry (cryptochrome) gene. Because the exact mechanisms of cryptochrome activity are unknown at the moment, researchers cannot quantify the impact of ELMFS on magnetoreception navigation, although many theorize that there is a negative impact.
What’s more is that birds also use a smell map in addition to their magnetic map. This smell map is dictated by a compound called dimethyl sulfide, which is produced by algae. Several species of pelagic birds use dimethyl sulfide as an indication of where the ocean is and more importantly, where food is. However, decaying plastic also emits dimethyl sulfide, which is why several birds consume plastic and end up clogging their gastrointestinal tract, leading to over a million seabirds dying each year.
Conclusion:
Avian magnetoreception is an intricate system of magnetite and cryptochrome activity that still baffles scientists today, and their ability to navigate thousands of miles away from home and back is an awe-inspiring ability. However, this ability is under threat due to anthropogenic sources of radiation and plastic. What makes this pollution even more of a menace is our current lack of comprehension as to how it might affect bird navigation. In order to preserve this magnificent sensory capability and save pelagic birds from toxic levels of plastic consumption, we must better understand the consequences of electromagnetic pollution and mitigate our plastic crisis.
References:
Image References:
Comments